What’s all the fuss about Regenerative Medicine?
Understanding Regenerative Medicine
Explore the concept of regenerative medicine and its role in healing various ailments using the body’s natural processes.
Benefits of Regenerative Medicine
Discover why patients are turning to regenerative medicine for non-invasive treatments with minimal side effects.
How Regenerative Medicine Works
Extraction and Application of Platelet-Rich Plasma (PRP)
Explain the process of obtaining PRP from a patient’s own blood or tissues and its application to promote healing.
Patient Experiences with Regenerative Medicine
Testimonials and Success Stories
Share real-life accounts of patients who have benefited from regenerative medicine treatments, highlighting their positive outcomes.
Future of Regenerative Medicine in Healthcare
Predictions and Trends
Discuss the potential growth and importance of regenerative medicine in future healthcare practices, likening its impact to groundbreaking medical advancements like penicillin.
Training and Implementation in Medical Practice
Integrating Regenerative Medicine
Explore how physicians are incorporating regenerative medicine into their practice through specialized training courses to enhance patient care.
Comparing Regenerative Medicine to Traditional Treatments
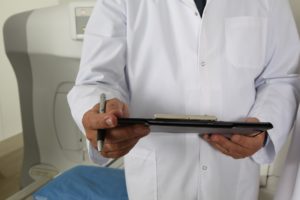
Advantages Over Conventional Medicine
Highlight the advantages of regenerative medicine over traditional medical approaches in terms of effectiveness and patient satisfaction.
Conclusion: Embracing Regenerative Medicine
Summarize the potential of regenerative medicine to revolutionize healthcare by offering effective, non-invasive treatments with fewer side effects.
References
Ensure comprehensive citations and references to support claims and information presented about regenerative medicine.
- Published in Blog
What’s True About Platelet-Rich Plasma For Stretch Marks?
MONDAY, 16 APRIL 2018 / PUBLISHED IN BLOG
Cindy is a career woman. When she became pregnant for the first time, she was torn between prioritizing her work and taking care of her growing body. To avoid drowning in that confusion, she kept herself busy with work all day while snacking during her free time. This led to rapid weight gain. Occasionally, her experienced sister would remind her to apply Bio Oil on her growing tummy before bed, but she was too exhausted to do it regularly. It wasn’t until after she delivered her baby that she realized her folly – her belly now looked like a road map.
What Works for Stretch Marks?
There are a variety of topical treatments, with those containing cocoa butter being trendy, but they hardly affect severe stretch marks. They perform better when used as preventive measures because fully developed stretch marks are rarely skin deep. The stretching occurs in the dermis layer underneath the surface. The inability of the epidermis to keep up with the stretching causes the appearance of deep roads of stretch marks.
One way to “cure” stretch marks, or at least reduce their appearance, is to make the skin surrounding the stretch marks more even with the stretch marks themselves. This can be achieved through various minimally invasive “scarring” technologies like microdermabrasion, microneedling, and CO2 fractional laser.
Platelet-Rich Plasma for Stretch Marks
How Platelet-Rich Plasma Works
Platelets can supply growth factors wherever healing is initiated. So unless healing is initiated or is still ongoing (which is not the case with fully developed stretch marks), the injected Platelet-Rich Plasma may not produce its excellent results.
That’s why in forums you can hear a lot of advice from doctors who claim Platelet-Rich Plasma can’t help stretch marks. In fact, that’d be the first thing I’d say if someone asked me. However, what if we could artificially initiate the healing? Not only in the outer epidermis layer, but also in the underlying dermis layer too? Now, that’s an excellent opportunity to put the growth factors in Platelet-Rich Plasma to good use, wouldn’t you agree?
PRP Microneedling for Stretch Marks
How PRP Microneedling Works
PRP microneedling involves swapping Vitamin C, used in traditional microneedling, with Platelet-Rich Plasma. This is traditionally called a Platelet-Rich Plasma facial because you’re essentially spreading blood components over your face. This is particularly effective for the face, but it can provide even better results for stretch marks.
Getting to the Root of the Problem
With microneedling, tiny holes are punched in both the outer epidermis layer and the inner dermis layer of the skin. These holes are so micro that they restore back to normal within minutes or hours. However, during the time they’re open, a healing response is triggered. Triggering a healing response in the inner dermis layer means there’s going to be some improvement in the stretch marks, as that’s where the source is. The procedure also removes unwanted, half-dead cells from the outer skin, causing the stretch marks to appear less deep.
Accelerated Healing with PRP
PRP accelerates the healing response triggered by the microneedles, and it must do so during the time the holes are open. So immediately after the microneedling, a concentrated gel of PRP is applied and massaged well enough for the platelets to seep through the holes. These platelets first stop the micro-bleeding caused by the microneedles, and then the growth factors in the platelets trigger the production of a substantial amount of collagen. Collagen’s primary role is the replacement of dead skin cells, which means it’ll replace all the dead, broken, and torn skin cells in the entire area. The result is fresh new skin in the areas of the stretch marks, causing them to shrink in size and look more rejuvenated.
Why Platelet-Rich Plasma?
The Healing Power of PRP
Platelet-Rich Plasma is a powerful healing component, initially discovered in 1987. Surgeons found that autologous platelet-rich plasma and red blood cell concentrates diminish the cost of healing for cardiac surgery, meaning faster, efficient, and natural healing for patients. The same force that heals cardiac surgery can also rejuvenate our skin or any other organ in the body. We’re only beginning to uncover the healing potential found in Platelet-Rich Plasma. Studies show that PRP can even heal bones.
PRP vs. Topical Products
It’d be foolish not to use such a potent, natural healing agent for skin rejuvenation purposes. Microneedling seems to be just what Platelet-Rich Plasma needs to exercise its healing powers. It’s much better than stockpiling topical products that might “cure” stretch marks, such as scar creams, retinoids, and peptides.
The Sooner, the Better
Studies show platelets are more efficient when introduced right after wound initiation. The same applies to stretch marks. As soon as you see those marks, it’s better to head straight to the clinic and get a Platelet-Rich Plasma + microneedling session to heal them. The longer you wait, the harder it gets to remove them. So stop experimenting with topical creams—they’re meant to be used as preventive measures.
- Published in Blog